Energy has been the cornerstone of the development and progress of human civilization over the years. It is the key to power, one of the most critical issues of the future, and has profound implications for the socio-economic-political sphere of humanity.
It has been a decisive factor in international affairs. Today, the increasing population and industrialization in this context have significantly raised energy needs. While the global primary energy consumption was 5,652.78 terawatt-hours (TWh) in 1800, it reached 157,063.77 TWh as of 2018.
Most of the primary energy consumed by the planet in 2018 was obtained by burning nonrenewable and rapidly depleting fossil fuels [1]. Considering the decrease in fossil fuel reserves, the difficulty of processing and reaching the reserves, and the adverse effects on the environment, renewable energy sources have become even more vital.
Along with the development of
technology, causing problems such as global climate change and environmental pollution, based on the use of energy sources from depleting raw materials over time, research and development of methods related to the use of renewable energy sources are increasing day by day.
The history of the Reverse Electrodialysis (RED) method, a renewable energy source, is based on the membrane accumulator system implemented by Manecke [2] in 1952. It is thought that his study inspired the first theoretical approaches that contributed to the idea of power generation by utilizing the salinity gradient difference in the future.
Salinity gradient energy is the energy obtained from the difference in salt concentration
between fresh water and salt water. The study, considered the first strategy of RED investigation, was conducted by Pattle [3] two years after Manecke’s study.
During the passage of fresh and saltwater through the membrane module called “hydroelectric battery”, an electromotive force is formed as a result of selective separation of ions by the series-connected anion exchange membranes (AEM) and cation exchange membranes (CEM) [3].
Figure 1 shows the flowchart of a standard RED system [4]. A typical RED system includes pumps, electrode solution, dilute and concentrated solution,
electrodes, spacers, gaskets, and ion exchange membranes.
The pumps circulate the concentrated and dilute solution as well as the electrode solution. Gaskets are used to prevent liquid solutions from leaking, and spacers allow ions to move freely.
RED is a system in which energy is produced from the ion transport mechanism via ion exchange membranes [5], [6]. Ion transport takes place through cation and anion exchange membranes arranged in rows. While positively charged ions pass through the CEM, negatively charged ions pass in the opposite direction through the AEM, thus
creating a positive and negative potential difference at the two ends, respectively [7].
The ionic current formed in the RED system is converted into electric current by the redox reactions taking place at the electrodes at the two ends (Figure 1) [8].
[caption id="attachment_143243" align="aligncenter" width="225"]
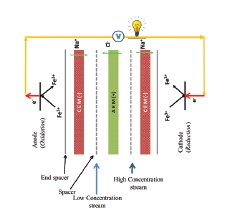
Figure 1. Schematic representation of a typical RED system [4][/caption]The basic logic of energy production and potential in the RED system is based on the 1st law of thermodynamics.This law is also known as the “conservation of energy”. Energy cannot be created out of nothing; existing energy cannot be destroyed either; it simply transforms from one form to another. [9].
Although the energy production in the RED system depends on the salt concentration of these waters, theoretically, 2.5 MJ of energy is expected to be generated by mixing 1 m3 of seawater with 1 m3 of fresh water, which is equivalent to the energy produced by a dam with a water height of approximately 250 m [10], [11].
Salinity gradient energy, also known as blue energy, has a huge potential of about 2.8 terawatts (TW) worldwide [12]. The worldwide RED potential is almost equivalent to the electrical energy currently consumed worldwide.
RED is a huge renewable energy source that does not negatively impact the environment
and does not pollute or emit carbon. It is directly related to the earth’s dynamic water cycle and depends on the energy dissipated when solutions with two different salt concentrations interact [12]–[14].
There are some reasons why this potential cannot be used today. The main reason is the use of membranes prepared for electrodialysis devices in the RED system.
These membranes are thought to negatively affect energy production with the salinity gradient [15]. Features such as membrane thickness, RED system design, space between membranes, and power density also significantly affect the system [16]–[18].
One of the results obtained in the work done by Pattle in the 19th century is that the membrane internal resistance is higher and the power output is lower at low temperatures.
In other words, the RED system is predicted to be more suitable in climates where seasonal
temperature differences are not large, and the water temperature is relatively higher [3].
While evaluating the potential of the RED system, it is necessary not to limit it to rivers as freshwater sources and the sea and oceans as saltwater sources. Although the spillage of rivers into seas, oceans, or similar water sources has significant potential, treated wastewater can also be used as a dilute water source in this system, while solutions in desalination facilities can be used as saltwater sources.
Recently, interest in these alternative feedwater trials has also increased. This situation is also critical within the scope of reusability of treated wastewater. Today, treatment plants in many coastal cities discharge their wastewater into the sea after treatment. One of the
best examples of this subject is Istanbul.
Istanbul is one of the most crowded metropolises in the world, with a population of more than 15 million. According to the annual report published by Istanbul Water and Sewerage Administration (İSKİ) in 2021, the annual amount of wastewater treated in wastewater treatment plants in Istanbul is 1,498,276,404 m3, while 608,660,129 m3 of this wastewater is treated biologically and advanced biological processes.
In the same report, the annual electricity consumed in wastewater treatment plants is specified as 380,068,959 kWh, while the annual electricity produced in these plants is stated as 146,769,893 kWh (URL-1).
While the data for Istanbul alone shows that a significant and clean energy source is wasted [19], similar observation is considered worldwide. An important issue here is the treatment of wastewater to reach a good quality effluent.
For this reason, biological and advanced biological treatments increase the function of these processes while minimizing the damage to the environment. The number of laboratory and pilot-scale studies in the literature on the RED system is increasing daily.
Generally, these studies are aimed at new module and membrane designs, process optimization, and minimizing the difficulties of the process. The first pilot-scale study was carried out in Italy in 2014 with a system with 125 membrane pairs. A maximum power density of 1.3 W/ m2 was obtained in the study carried out with seawater and saturated brine samples [20], [21].
In 2014, the world’s first RED power plant was established in the Netherlands in partnership with Wetsus, Fujifilm and RedStack. In this facility, where custom-made membranes are used, 50 kW of electricity was produced by using IJsselmeer water (freshwater) located near Afsluitdijk, the largest dam in the Netherlands, and Wadden
Sea Water (saltwater).
Researchers have stated that this power plant has an enormous power generation potential
to meet the energy needs of 500,000 households (URL-2).Studies are underway to ensure that RED facilities do not only generate electricity but also directly become a fuel cell-based power source.
The most important of these was the high-efficiency hydrogen production by utilizing the salinity gradient difference between seawater and treated wastewater in a pilot-scale facility in Fukuoka, Japan, where hydrogen production was carried out using RED. Thus, it was observed that the salinity gradient difference can be directly used for efficient gas fuel generation for fuel cell-based power supply.
The system that combines RED with electricity and hydrogen production is called RED-H2. One of the promising solutions for constraints such as the irregularity of the energy produced from renewable sources and the inability to store the excess electricity production is the “Power to Gas (P2G)” technology.
With P2G technology, electrical energy is converted into gases such as hydrogen or methane, and stored and used when needed [22]–[24].
The demonstration of the usability of reverse electrodialysis technology for hydrogen production with the RED-H2 system is fascinating in this sense. The geographical location of Turkey allows real-scale studies to be carried out.
Turkey is surrounded by the Black Sea in the North, the Marmara Sea in the Northwest,
the Aegean Sea in the West and the Mediterranean in the South. In addition, the waters of some lakes, such as Salt Lake and Burdur Lake in Turkey, are as salty as seawater.
Among the top 20 rivers with the highest energy density (energy potential per cubic meter of freshwater) in the world, Büyük Menderes ranks first and Ceyhan River ranks third.
However, in addition to a high energy density, a stable salinity gradient difference and a high achievable energy value are required [25]. It should be noted that obtaining the desired size of energy from the system depends on more than one factor, such as providing
suitable operating conditions and system efficiency.
The Mediterranean sea stands out because the water salinity and average temperatures are high enough to affect the RED system performance positively. It is belived that Turkey has a significant and advantageous geographical position in terms of the application of RED technology.
RED technology can be applied on an industrial scale with the use of concentrated brine, or at the discharge points of wastewater treatment plant effluent into the sea, in locations where river basins flow into the sea in Turkey, and it has a promising potential in terms of increasing the use of local resources in energy production.
However, it is essential to determine the energy production potential with studies that reflect different environmental conditions on a regional scale.
As a result, the salinity gradient energy is the clean energy produced by the chemical energy resulting from mixing two solutions of different salinity. Utilizing this renewable energy potential can contribute to responding to the country’s energy deficit.
RED has significant potential to contribute to realizing the aim of meeting Turkey’s energy needs with socially, economically and environmentally appropriate methods.
Reference
[1] A. Zoungrana ve M. Çakmakci, “From non-renewable energy to renewable by harvesting salinity gradient power by reverse electrodialysis: A review”, International Journal of Energy Research, c. 45,
sayı 3. 2021.
[2] G. Manecke, “Membranakkumulator”, Zeitschrift für Phys. Chemie, c. 201, sayı 1, ss. 1–15, 1952.
[3] R. E. Pattle, “Production of Electric Power by mixing Fresh and Salt Water in the
Hydro- electric”, Nature, c. 174, s. 660, 1954.
[4] R. Ortiz-Imedio, L. Gomez-Coma, M. Fallanza, A. Ortiz, R. Ibañez, ve I. Ortiz, “Comparative performance of Salinity Gradient Power-Reverse Electrodialysis under different operating conditions”, Desalination, c. 457, ss. 8–21, 2019 .
[5] D. Kim, K. Kwon, D. H. Kim, ve L. Li, Energy Generation Using Reverse Electrodialysis: Principles, Implementation, and Applications. 2019.
[6] D. A. Vermaas, Energy generation from mixing salt water and fresh water. 2014.
[7] D. A. Vermaas, E. Guler, M. Saakes, ve K. Nijmeijer, “Theoretical power density from salinity
gradients using reverse electrodialysis”, Energy Procedia, c. 20, ss. 170–184, 2012.
[8] J. Veerman, M. Saakes, S. J. Metz, ve G. J. Harmsen, “Reverse electrodialysis: A validated process model for design and optimization”, Chem. Eng. J., c. 166, sayı 1, ss. 256–268, 2011.
[9] J. N. Weinstein ve F. B. Leitz, “Electric power from differences in salinity: The dialytic battery”, Science (80-. )., c. 191, sayı 4227, ss.557–559, 1976.
[10] J. W. Post vd., “Salinity-gradient power: Evaluation of pressure-retarded osmosis and reverse electrodialysis”, J. Memb. Sci., c. 288, sayı 1–2, ss. 218–230, 2007.
[11] K. Nijmeijer ve S. Metz, “Chapter 5 Salinity Gradient Energy”, Sustainability Science and Engineering, c. 2, sayı C. 2010.
[12] J. Veerman, “Reverse Electrodialysis: design and optimization by modeling and experimentation”,
2010.
[13] S. Pawlowski, J. Crespo, ve S. Velizarov, “Sustainable power generation from salinity gradient energy by reverse electrodialysis”, içinde Electrokinetics Across Disciplines and Continents:
New Strategies for Sustainable Development, 2015.
[14] J. G. Hong, W. Zhang, J. Luo, ve Y. Chen, “Modeling of power generation from the mixing of simulated saline and freshwater with a reverse electrodialysis system: The effect of monovalent and multivalent ions”, Appl. Energy, c. 110, ss. 244–251, 2013.
[15] A. H. Avci vd., “Sulfonated polyethersulfone based cation exchange membranes for
reverse electrodialysis under high salinity gradients”, J. Memb. Sci., c. 595, s. 117585, 2020.
[16] D. A. Vermaas, M. Saakes, ve K. Nijmeijer, “Doubled power density from salinity gradients at reduced intermembrane distance”, Environ. Sci. Technol., c. 45, sayı 16, ss. 7089–7095, 2011.
[17] N. Y. Yip, D. A. Vermaas, K. Nijmeijer, ve M. Elimelech, “Thermodynamic, energy efficiency, and power density analysis of reverse electrodialysis power generation with natural salinity gradients”, Environ. Sci. Technol., c. 48, sayı 9, ss. 4925–4936, 2014.
[18] H. K. Kim, M. S. Lee, S. Y. Lee, Y. W. Choi, N. J. Jeong, ve C. S. Kim, “High power density of reverse electrodialysis with pore-filling ion exchange membranes and a high-open-area spacer”, J. Mater. Chem. A, c. 3, sayı 31, ss. 16302–16306, 2015.
[19] A. Zoungrana, O. K. Türk, ve M. Çakmakci, “Energy coverage of ataköy-ambarlı municipal wastewater treatment plants by salinity gradient power”, J. Water Process Eng., c. 38, sayı July, s. 101552, 2020.
[20] H. Tian, Y. Wang, Y. Pei, ve J. C. Crittenden, “Unique applications and improvements of reverse electrodialysis: A review and outlook”, Applied Energy, c. 262. 2020.
[21] M. Tedesco, C. Scalici, D. Vaccari, A. Cipollina, A. Tamburini, ve G. Micale, “Performance of the first reverse electrodialysis pilot plant for power production from saline waters and concentrated brines”, J. Memb. Sci., c. 500, ss. 33–45, 2016.
[22] M. Higa vd., “Sustainable hydrogen production from seawater and sewage treated water using reverse electrodialysis technology”, Water Pract. Technol., c. 14, sayı 3, ss. 645–651, 2019.
[23] M. Götz vd., “Renewable Power-to-Gas: A technological and economic review”, Renew. Energy, c. 85, ss. 1371–1390, 2016.
[24] G. Gahleitner, “Hydrogen from renewable electricity: An international review of power-to-gas pilot plants for stationary applications”, Int. J. Hydrogen Energy, c. 38, sayı 5, ss. 2039–2061, 2013.
[25] O. A. Alvarez-Silva, A. F. Osorio, ve C. Winter, “Practical global salinity gradient energy potential”, Renew. Sustain. Energy Rev., c. 60, ss. 1387–1395, 2016.
URL-1 İstanbul Su ve Kanalizasyon İdaresi (İSKİ), ‘’2021 yılı Faaliyet Raporu’’, https://www.iski.gov.tr/web/assets/video/Genel%20Kurul%20Konuşması/2021%20FAALİYET%20RAPORU.pdf
URL-2 Dutch Water Sector, “Dutch King Opens World’s First RED Power Plant Driven on Fresh-Salt Water Mixing”, https://www.dutchwatersector.com/news/dutch-king-opens-worlds-first-red-powerplant-driven-on-fresh-salt-water-mixing (2014)
Prof. Dr. Mehmet Çakmakcı
Yildiz Technical University
Faculty of Civil Engineering
Department of Environmental
Engineering
Dr. Ali Zoungrana
University of Manitoba
Price Faculty of Engineering
Department of Civil Engineering
Environmental Engineering
Arş. Gör. Oruç Kaan Türk
Yildiz Technical University
Faculty of Civil Engineering
Department of Environmental
Engineering